Affordable + Large-scale [Metal → Composite]
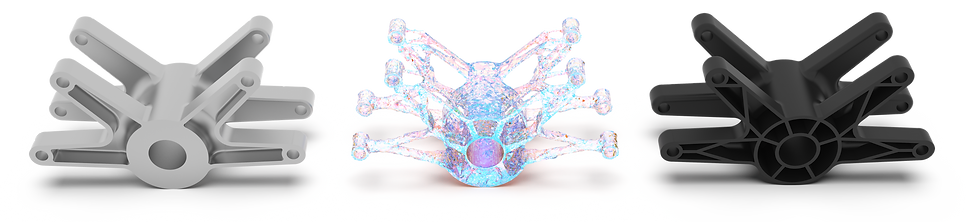
Original (die-cast aluminum)
1,080 g
SFTC Part
427 g
AI-driven Topology
60% Weight Reduction
Reinforced thermoplastics are ubiquitous in non-structural / semi-structural applications, but replacement of metals in demanding applications have been challenging until now. Thanks to AirGo’s proprietary short-fiber thermoplastic composite (SFTC) technology, we are able to offer affordable lightweighting solutions that were not previously possible due to commercial, production or technical limitations.

Let’s get to know your requirements…
Geometry
Surface Finish
Weight Target
Flammability
Max Deflection
Operating Temperature
Interface with other parts
Tolerance
Food Grade
Corrosion
Biocompatibility
Renewable Source
Abrasion Resistance
EMI/RFI
Electrical Conductivity
Impact
Thermal Conductivity
Structural Loads
Humidity
Cost Target
Chemical Compatibility
Sterilization
Fatigue Life
Color
Wear
Step 1: AI-driven Technology



Reinforced thermoplastics are ubiquitous in non-structural / semi-structural applications, but replacement of metals in demanding applications have been challenging until now. Thanks to AirGo’s proprietary short-fiber thermoplastic composite (SFTC) technology, we are able to offer affordable lightweighting solutions that were not previously possible due to commercial, production or technical limitations.
Step 2: Non-linear Structural Analysis



Unlike metals, reinforced thermoplastics undergo strain hardening within their visco-elastic limit. This means that the material behavior at elevated stress levels is highly non-linear. Other non-linear effects such as large displacement and complex contacts must be considered too. We then use implicit static or explicit dynamic non-linear solver with strain-rate-dependence to accurately simulate the behavior of the part.
Step 3: Fiber Superficial Flow Simulation
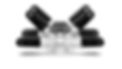
Computational fluid dynamics is used to determine how the fibers flow in the mold and where the weld surfaces are formed during injection. The mold design then undergoes several round of optimization to determine the best configuration of gates. The effects of cooling channels, injection speed, mold temperature, gate diameter and opening sequence as well as packaging pressure are also analyzed.
Step 4: AirGo’s proprietary micromechanics
Accurate stiffness and damage prediction at no extra computational cost

The failure behavior of metals and traditional composite laminates are described by well-known models (e.g. Von Mises and Hashin’s criteria, respectively). Fracture mechanics in injection molded composites is far more complicated. In academia, the stiffness of a part is determined using millions of RVEs (representative volume elements) based on the Mori-Tanaka model. This method is very time-consuming, requires clusters of supercomputers and is only applicable to models with 3D mesh. In contrast, AirGo’s proprietary model uses homogenized properties that are calculated from flow and cross-flow curves and therefore do not require any extra computing power. The damage model, which is based on element-level coordinates that dictate strain-based failure in the matrix, can be applied during post-processing and is therefore independent of the solver or the mesh used.
Step 5: Fatigue & Creep Analysis

If the component is expected to remain in operation for many cycles, we perform detailed fatigue and creep analysis taking into account variables such as stress amplitude and magnitude, number of cycles or time, temperature and moisture absorption among others. AirGo has experience developing solutions for demanding applications with 150+ million cycles.
Step 6: Precision Tooling & Production

Once the design phase is finalized, it is important to make sure that actual part can satisfy the requirements it was designed for. AirGo has developed a rigorous aerospace-grade QC system that relies on extensive statistical data as well as validation tests. Our partner network includes carefully-selected ODMs that are trained to properly use our technology. We also perform frequent audits to make sure that every batch of parts that you receive meets the same level of quality that you have been promised.
Step 7: Testing & Validation
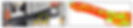
While our simulations are highly accurate, we understand that certain industries require physical testing. Upon request, we are able to carry out a wide range of tests to ensure the performance of the part exceeds all your structural, physical and chemical requirements.